Professor Peter Horton FRS
School of Biosciences
Emeritus Professor of Biochemistry
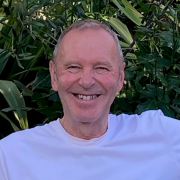
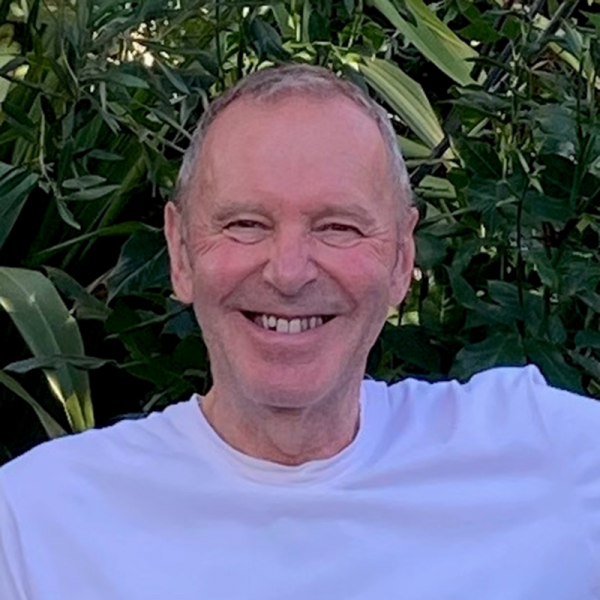
Full contact details
School of Biosciences
Firth Court
Western Bank
Sheffield
S10 2TN
- Profile
-
- 2008 - present: Emeritus Professor of Biochemistry, School of Biosciences, University of Sheffield
- 2014 - 2019: Chief Research Advisor, Grantham Centre for Sustainable Futures, University of Sheffield
- 2008 - 2014: Research Advisor, Project Sunshine, Faculty of Science, University of Sheffield
- 1990 - 2008: Professor of Plant Biochemistry, University of Sheffield
- 1989 - 2003: Chairman, Robert Hill Institute, University of Sheffield
- 1978 - 1990: Lecturer/Reader, Department of Molecular Biology and Biotechnology University of Sheffield
- 1975 - 1978: Assistant Professor, Department of Cell and Molecular Biology, State University of New York at Buffalo, USA
- 1973 - 1975: Postdoctoral Research Associate, Department of Biological Sciences, Purdue University, USA
- 1970 - 1973: D.Phil student, Department of Biology, University of York
- Research interests
-
Interdisciplinary approaches to sustainable food security
Food is a basic human need and its production and consumption are embedded in all aspects of our society. Food Security has been defined as a situation that exists when all people, at all times, have physical, social and economic access to sufficient, safe and nutritious food that meets their dietary needs and food preferences for an active and healthy life. Characteristics of achieving food security include: high agricultural productivity; stability to changes in weather and markets; resilience to stresses and shocks; and equity in supply. Sustainability is a term also broadly set out, as meaning meeting the needs and aspirations of the present without compromising the ability of future generations to meet theirs. Therefore Sustainable Food Security means reconciliation of the frequently conflicting environmental, social equity and economic demands. Successful research aimed at reaching this goal is unlikely to be confined within single disciplines but will inevitably have to integrate many, in science, engineering, social science and medicine. Whilst enhanced agricultural production with new technology is a pre-requisite it will not on its own be sufficient. The challenge is how to integrate research across disciplines such that farmers, agri-food industries, policy makers and consumers have the evidence upon which to make the best decisions and interventions.
1. Delivering sustainable food security: analysis of agri-food ecosystems
It is now widely accepted that our food supply systems are in crisis, whether on the national or global scale. The implementation of an agri-tech strategy takes place in the context of a food supply system in crisis. Disruptions in supply due to extreme weather events, over-reliance on scarce or expensive inputs, increased energy costs, increasing demand from an increasing population, major contributions to greenhouse gas emissions, changing food consumption patterns, growing number of links between human health and the food we eat, and the increased complexity of global food supply chains are some of the factors involved. Current systems and practices not only deliver insufficient food but are economically and environmentally unsustainable, and risk social upheaval, political unrest and a human health disaster.
Achieving adequate food production, ensuring environmental sustainability and promoting human health, impinge on all aspects of the food supply chain. Despite these constraints, farmers, producers and retailers have to retain adequate profit margins whilst food prices stay within the budgets of the consumer. However, forming effective business strategies, coherent agrifood policies and appropriate research and development programmes across such a complex system presents major challenges. How do we take the right actions in the right place at the right time? How do we optimise such actions for maximum benefit and desired outcome? How do we decide which are the most desirable outcomes? Inevitably there will be trade-offs, throughout the food supply chain. eg high yield potential of a crop variety may be incompatible with maximum stress tolerance; maximum levels of production may not be environmentally sustainable; low food prices may be inconsistent with farm profitability or high nutritional value. Making decisions about what trade-offs are practical and acceptable is the critical challenge we face and needs a multidisciplinary effort in which scientists, engineers and social scientists work together in collaboration with farmers, food producers, retailers and consumers to provide specific and practical solutions. Such an unprecedented level of integration in the agri-food sector is a significant challenge.
Extension of the agroecological approach to encompass the entire food production and consumption chain is a promising way forward. Such an agri-food ecosystem would have four key elements: agricultural and land use strategy; crop production and harvesting; processing, storage and distribution; retailing and consumption, and would require:
1. Description of all the people, components and processes within a unified internally consistent framework
2. Analysis to understand how it evolved (by selection or design) and how it works (dynamics, feedbacks)
3. Systems approaches to quantify it in a transparent accessible form
4. Simple models to predict outcomes under a range of scenariosWaste and losses can then be identified and measured together with all of the environmental and social penalties. The impact of actions of key stakeholders (landowners, farmers, agricultural centres, agrifood industry, distributers, traders, consumers) and the external factors (governments, international agencies, markets, lobbyists; NGOs; science and technology, education, media, culture) can all be assessed.
It is only by reaching this level of analysis and understanding can we hope to implement the right innovation at the right time in the right place. The effects of intervention and change could be predicted and key questions answered. eg What might be the effect of a change in a particular aspect of consumer choice on crop production and resource use. What would be the implications for the food producer, retailer and consumer of a change to a more sustainable and resilient crop production, through a new plant variety or agronomic practice. Where are the pressure points or sites of greatest sensitivity to change? Where are the hotspots in terms of resource use, environmental effects or waste? How do we adapt to climate change? What are the barriers to uptake of new technologies? How can we accommodate the huge diversity of crops and farms?
2. Optimisation of photosynthesis for increasing crop yield
To provide more crop yield on less land with fewer inputs undoubtedly requires alteration to the fundamental physiological attributes of plants. Included in these is the increase in efficiency of photosynthesis. Many approaches are being taken to achieve this goal, most of which involve alteration of parts of the basic photosynthetic machinery. An alternative approach arises from observation made on rice photosynthesis, done in collaboration with the International Rice Research Institute. This work revealed some striking insights, mainly how poor photosynthesis was in the field, even under conditions widely regarded as optimum. The conclusion from this study is important: there is enough photosynthetic activity in the existing cellular machinery to sustain a much larger yield if only plants could be induced to perform at their full potential. So why don’t plants perform at their full potential? One reason why photosynthetic activity is not maximally expressed could be inappropriate optimisation. Put simply, stability and survival (a low risk strategy) in the natural environment are driving forces of evolution, not necessarily high growth rate and photosynthetic rate (a high risk strategy) even though there is potential for high grain yield. Photosynthesis is held back below its potential because growth is optimised in the face of the particular properties of the plant’s habitat. Therefore, we have to consider the evolution and basic biology of each crop species.
Particularly important is that the environment is never constant- there are fluctuations in levels of sunlight, temperature and rainfall. Plants record, memorise and (try to) predict their environments to ensure that they always have enough energy storage from photosynthesis to power their growth and development. For example, plants have to determine the size of their reproductive sinks (i.e. grain capacity) in advance, predicting what the photosynthetic rate will be to give maximum grain filling. Over-estimation of future photosynthesis results in poor grain filling and/or poor quality grain; under-estimation of future photosynthesis results in a decrease in the efficiency of solar energy use and losses of potential productivity. Trade-offs inevitably result from optimisation of the internal regulatory mechanisms involved (dynamic range, kinetics, precision), and this readily explains the apparent under-performance of photosynthesis. Consequently, there may be opportunities for the breeding of higher yielding crops by tailoring regulatory responses to specific agricultural scenarios, where man’s intervention has moderated some of the environmental constraints on productivity, by irrigation, provision of fertilisers and elimination of weeds. A key point is that optimisation will vary according to plant species or variety, the climate and season, the agronomic practice, the locality and so on. Thus, significant benefits will come from understanding at the molecular and genetic levels how to alter the optimisation of the biochemistry and physiology of individual leaves, their performance in the whole plant, and the way individual plants interact in the crop canopy. Indeed, such knowledge may also be necessary to offset the inherent conservatism of plants that could thwart current attempts to increase photosynthetic efficiency, and hence yield, by manipulation of with the basic biochemical processes of carbon assimilation.
3. Understanding photoprotection in plants
Molecular transitions regulate the structure and function the photosynthetic membrane in order to adapt its function to different environmental and metabolic conditions. Multidisciplinary investigations have provided insights into these transitions, combining biochemical and structural analysis of purified proteins with spectroscopic, physiological and genetic analyses of intact systems. In order to maximise their use of light energy in photosynthesis, plants have light-harvesting antennae, which collect light quanta and deliver them to the reaction centres, where energy conversion takes place. The functioning of the antenna responds to the changes in the intensity of sunlight encountered in nature. In shade, light is efficiently harvested in photosynthesis. However, in full sunlight or whenever there is a restriction on the metabolic demand for photosynthetic product (eg in cold conditions), much of the energy absorbed is not needed and vitally important switches to specific antenna states safely dissipate the excess energy as heat. This is essential for plant survival because it provides protection against the potential photo-damage of the photosynthetic membrane. The understanding the molecular mechanisms involved in photoprotection has the potential to be exploited to produce crops plant better adapted to harsh environmental and climatic conditions.
- Publications
-
Journal articles
- A sustainable food future. Royal Society Open Science, 10(8).
- Technologies to deliver food and climate security through agriculture.. Nat Plants, 7(3), 250-255.
- COVID-19 and the climate emergency: Do common origins and solutions reside in the global agrifood system?. One Earth, 3(1), 20-22.
- Re-defining sustainability : living in harmony with life on Earth. One Earth, 1(1), 86-94. View this article in WRRO
- Food chain inefficiency (FCI) : accounting conversion efficiencies across entire food supply chains to re-define food loss and waste. Frontiers in Sustainable Food Systems, 3. View this article in WRRO
- Joan Mary Anderson 1932–2015. Historical Records of Australian Science, 30(1), 19-19.
- Joan Mary Anderson. 12 May 1932—28 August 2015. Biographical Memoirs of Fellows of the Royal Society, 65, 7-29.
- Integrating evidence, politics and society: a methodology for the science–policy interface. Palgrave Communications, 4(1). View this article in WRRO
- Why rational argument fails the genetic modification (GM) debate. Food Security, 10(5), 1145-1161. View this article in WRRO
- We need radical change in how we produce and consume food. Food Security, 9(6), 1323-1327. View this article in WRRO
- An agenda for integrated system-wide interdisciplinary agri-food research. Food Security, 9(2), 195-210. View this article in WRRO
- The environmental impact of fertilizer embodied in a wheat-to-bread supply chain. Nature Plants, 3. View this article in WRRO
- Remembering Joan (Jan) Mary Anderson (1932–2015). Photosynthesis Research, 129(2), 129-146.
- Fingerprinting the macro-organisation of pigment-protein complexes in plant thylakoid membranes in vivo by circular-dichroism spectroscopy. Biochimica et Biophysica Acta (BBA) - Bioenergetics, 1857(9), 1479-1489.
- An integrated theoretical framework to enhance resource efficiency, sustainability and human health in agri-food systems. Journal of Cleaner Production, 120, 164-169. View this article in WRRO
- An intact light harvesting complex I antenna system is required for complete state transitions in Arabidopsis. Nature Plants, 1(12). View this article in WRRO
- David Alan Walker. 18 August 1928 — 13 February 2012. Biographical Memoirs of Fellows of the Royal Society, 60, 413-432.
- The Specificity of Controlled Protein Disorder in the Photoprotection of Plants. Biophysical Journal, 105(4), 1018-1026.
- Optimization of light harvesting and photoprotection: molecular mechanisms and physiological consequences. Philosophical Transactions of the Royal Society B: Biological Sciences, 367(1608), 3455-3465.
- The photoprotective protein PsbS exerts control over CO2 assimilation rate in fluctuating light in rice. The Plant Journal, no-no.
- Preface. Philosophical Transactions of the Royal Society B: Biological Sciences, 367(1608), 3381-3383.
- Controlled Disorder in Plant Light-Harvesting Complex II Explains Its Photoprotective Role. Biophysical Journal, 102(11), 2669-2676.
- Towards elucidation of dynamic structural changes of plant thylakoid architecture. Philosophical Transactions of the Royal Society B: Biological Sciences, 367(1608), 3515-3524.
- Impacts of long-term enhanced UV-B radiation on bryophytes in two sub-Arctic heathland sites of contrasting water availability. Annals of Botany, 108(3), 557-565.
- Molecular Adaptation of Photoprotection: Triplet States in Light-Harvesting Proteins. Biophysical Journal, 101(4), 934-942.
- Impact of chlororespiration on non-photochemical quenching of chlorophyll fluorescence and on the regulation of the diadinoxanthin cycle in the diatom Thalassiosira pseudonana. Journal of Experimental Botany, 62(2), 509-519.
- The PsbS protein controls the macro-organisation of photosystem II complexes in the grana membranes of higher plant chloroplasts. FEBS Letters, 584(4), 759-764.
- Effect of xanthophyll composition on the chlorophyll excited state lifetime in plant leaves and isolated LHCII. Chemical Physics, 373(1-2), 23-32.
- Agriculture and the new challenges for photosynthesis research. New Phytologist, 181(3), 532-552.
- Comparison of the Thermodynamic Landscapes of Unfolding and Formation of the Energy Dissipative State in the Isolated Light Harvesting Complex II. Biophysical Journal, 97(4), 1188-1197.
- The Zeaxanthin-Independent and Zeaxanthin-Dependent qE Components of Nonphotochemical Quenching Involve Common Conformational Changes within the Photosystem II Antenna in Arabidopsis. Plant Physiology, 149(2), 1061-1075.
- The Photosystem II Light-Harvesting Protein Lhcb3 Affects the Macrostructure of Photosystem II and the Rate of State Transitions in Arabidopsis. The Plant Cell, 21(10), 3245-3256.
- The role of lutein in the acclimation of higher plant chloroplast membranes to suboptimal conditions. Physiologia Plantarum, 134(1), 227-236.
- The PsbS Protein Controls the Organization of the Photosystem II Antenna in Higher Plant Thylakoid Membranes. Journal of Biological Chemistry, 283(7), 3972-3978.
- The xanthophyll cycle pool size controls the kinetics of non-photochemical quenching inArabidopsis thaliana. FEBS Letters, 582(2), 262-266.
- The Lhcb protein and xanthophyll composition of the light harvesting antenna controls the ΔpH-dependency of non-photochemical quenching inArabidopsis thaliana. FEBS Letters, 582(10), 1477-1482.
- Photosynthetic acclimation: Does the dynamic structure and macro-organisation of photosystem II in higher plant grana membranes regulate light harvesting states?. FEBS Journal, 275(6), 1069-1079.
- Induction of Efficient Energy Dissipation in the Isolated Light-harvesting Complex of Photosystem II in the Absence of Protein Aggregation. Journal of Biological Chemistry, 283(43), 29505-29512.
- Bryophyte physiological responses to, and recovery from, long-term nitrogen deposition and phosphorus fertilisation in acidic grassland. New Phytologist, 180(4), 864-874.
- Elevated Zeaxanthin Bound to Oligomeric LHCII Enhances the Resistance ofArabidopsisto Photooxidative Stress by a Lipid-protective, Antioxidant Mechanism. Journal of Biological Chemistry, 282(31), 22605-22618.
- Identification of a mechanism of photoprotective energy dissipation in higher plants. Nature, 450(7169), 575-578.
- Lack of the Light-Harvesting Complex CP24 Affects the Structure and Function of the Grana Membranes of Higher Plant Chloroplasts. The Plant Cell, 18(11), 3106-3120.
- Plasticity in the Composition of the Light Harvesting Antenna of Higher Plants Preserves Structural Integrity and Biological Function. Journal of Biological Chemistry, 281(21), 14981-14990.
- PsbS enhances nonphotochemical fluorescence quenching in the absence of zeaxanthin. FEBS Letters, 580(8), 2053-2058.
- Differential adaptation of two varieties of common bean to abiotic stress. Journal of Experimental Botany, 57(3), 685-697.
- Differential adaptation of two varieties of common bean to abiotic stress. Journal of Experimental Botany, 57(3), 699-709.
- Control of the light harvesting function of chloroplast membranes: The LHCII-aggregation model for non-photochemical quenching. FEBS Letters, 579(20), 4201-4206.
- Acclimation of photosynthesis to high irradiance in rice: gene expression and interactions with leaf development. Journal of Experimental Botany, 56(411), 449-460.
- Granal stacking of thylakoid membranes in higher plant chloroplasts: the physicochemical forces at work and the functional consequences that ensue. Photochemical & Photobiological Sciences, 4(12), 1081-1081.
- Entropy-assisted stacking of thylakoid membranes. Biochimica et Biophysica Acta (BBA) - Bioenergetics, 1708(2), 187-195.
- Molecular basis of photoprotection and control of photosynthetic light-harvesting. Nature, 436(7047), 134-137.
- Paraheliotropism can protect water-stressed bean (Phaseolus vulgaris L.) plants against photoinhibition. Journal of Plant Physiology, 161(12), 1315-1323.
- Arabidopsis AtCYP20-2 Is a Light-Regulated Cyclophilin-Type Peptidyl-Prolyl cis-trans Isomerase Associated with the Photosynthetic Membranes. Plant Physiology, 134(4), 1244-1247.
- The Arabidopsis Cyclophilin Gene Family. Plant Physiology, 134(4), 1268-1282.
- Acclimation of Arabidopsis thaliana to the light environment: the relationship between photosynthetic function and chloroplast composition. Planta, 218(5), 793-802.
- The Functional Significance of the Monomeric and Trimeric States of the Photosystem II Light Harvesting Complexes†. Biochemistry, 43(2), 501-509.
- A Mutant of Arabidopsis Lacking the Triose-Phosphate/Phosphate Translocator Reveals Metabolic Regulation of Starch Breakdown in the Light. Plant Physiology, 135(2), 891-906.
- Insights into the molecular dynamics of plant light-harvesting proteins in vivo. Trends in Plant Science, 9(8), 385-390.
- The super-excess energy dissipation in diatom algae: comparative analysis with higher plants. Photosynthesis Research, 82(2), 165-175.
- Thermodynamic Investigation into the Mechanism of the Chlorophyll Fluorescence Quenching in Isolated Photosystem II Light-harvesting Complexes. Journal of Biological Chemistry, 278(24), 21845-21850.
- Effects of season-dependent irradiance levels and nitrogen-deficiency on photosynthesis and photoinhibition in field-grown rice (Oryza sativa). Physiologia Plantarum, 117(3), 343-351.
- Plants lacking the main light-harvesting complex retain photosystem II macro-organization. Nature, 421(6923), 648-652. View this article in WRRO
- Identification of Mutants of Arabidopsis Defective in Acclimation of Photosynthesis to the Light Environment. Plant Physiology, 131(2), 472-481.
- Stark spectroscopy of the light-harvesting complex II in different oligomerisation states. Biochimica et Biophysica Acta (BBA) - Bioenergetics, 1605(1-3), 83-95.
- Decreased Content of Leaf Ferredoxin Changes Electron Distribution and Limits Photosynthesis in Transgenic Potato Plants. Plant Physiology, 133(4), 1768-1778.
- The Structure of Photosystem II inArabidopsis:Localization of the CP26 and CP29 Antenna Complexes†. Biochemistry, 42(3), 608-613.
- Absence of the Lhcb1 and Lhcb2 proteins of the light-harvesting complex of photosystem II - effects on photosynthesis, grana stacking and fitness. The Plant Journal, 35(3), 350-361.
- In vitro reconstitution of the activated zeaxanthin state associated with energy dissipation in plants. Proceedings of the National Academy of Sciences, 99(25), 16331-16335.
- Acclimation of Rice Photosynthesis to Irradiance under Field Conditions. Plant Physiology, 130(4), 1999-2010.
- Molecular Configuration of Xanthophyll Cycle Carotenoids in Photosystem II Antenna Complexes. Journal of Biological Chemistry, 277(45), 42937-42942.
- Are there associations between grain-filling rate and photosynthesis in the flag leaves of field-grown rice?. Journal of Experimental Botany, 53(378), 2217-2224.
- Overexpression of β-carotene hydroxylase enhances stress tolerance in Arabidopsis. Nature, 418(6894), 203-206. View this article in WRRO
- Linking drought‐resistance mechanisms to drought avoidance in upland rice using a QTL approach: progress and new opportunities to integrate stomatal and mesophyll responses. Journal of Experimental Botany, 53(371), 989-1004.
- Activation of Zeaxanthin Is an Obligatory Event in the Regulation of Photosynthetic Light Harvesting. Journal of Biological Chemistry, 277(10), 7785-7789.
- A Critical Role for the Var2 FtsH Homologue ofArabidopsis thalianain the Photosystem II Repair Cyclein Vivo. Journal of Biological Chemistry, 277(3), 2006-2011.
- Light-Induced Trimer to Monomer Transition in the Main Light-Harvesting Antenna Complex of Plants: Thermo-Optic Mechanism†. Biochemistry, 41(51), 15121-15129.
- Acclimation of Arabidopsis thaliana to the light environment: the existence of separate low light and high light responses. Planta, 213(5), 794-801.
- Kinetic Analysis of Nonphotochemical Quenching of Chlorophyll Fluorescence. 2. Isolated Light-Harvesting Complexes†. Biochemistry, 40(33), 9902-9908.
- Kinetic Analysis of Nonphotochemical Quenching of Chlorophyll Fluorescence. 1. Isolated Chloroplasts†. Biochemistry, 40(33), 9896-9901.
- Configuration and Dynamics of Xanthophylls in Light-harvesting Antennae of Higher Plants. Journal of Biological Chemistry, 276(27), 24862-24870.
- Antisense Inhibition of the Photosynthetic Antenna Proteins CP29 and CP26: Implications for the Mechanism of Protective Energy Dissipation. The Plant Cell, 13(5), 1193-1204.
- Allosteric regulation of the light-harvesting system of photosystem II. Philosophical Transactions of the Royal Society of London. Series B: Biological Sciences, 355(1402), 1361-1370.
- Chlorophyll fluorescence quenching in isolated light harvesting complexes induced by zeaxanthin. FEBS Letters, 471(1), 71-74.
- Prospects for crop improvement through the genetic manipulation of photosynthesis: morphological and biochemical aspects of light capture. Journal of Experimental Botany, 51(suppl_1), 475-485.
- C4 photosynthesis in rice: some lessons from studies of C3 photosynthesis in field-grown rice**Citation: Sheehy JE, Mitchell PL, Hardy B, editors. 2000. Redesigning rice photosynthesis to increase yield. Proceedings of the Workshop on The Quest to Reduce Hunger: Redesigning Rice Photosynthesis, 30Nov.-3 Dec. 1999, Los Baños. Philippines. Makati City (Philippines): International Rice Research Institute and Amsterdam (The Netherlands): Elsevier Science B.V. 293 p., 127-144.
- . Photosynthesis Research, 64(1), 1-13.
- Pigment Binding Site Properties of Two Photosystem II Antenna Proteins. Journal of Biological Chemistry, 275(29), 22031-22036.
- Acclimation of Arabidopsis thaliana to the light environment: the role of photoreceptors. Planta, 209(4), 517-527.
- Chloroplast Acclimation in Leaves of Guzmania monostachia in Response to High Light. Plant Physiology, 121(1), 89-96.
- Spectroscopic characterization of the spinach Lhcb4 protein (CP29), a minor light-harvesting complex of photosystem II. European Journal of Biochemistry, 262(3), 817-823.
- Determination of the Stoichiometry and Strength of Binding of Xanthophylls to the Photosystem II Light Harvesting Complexes. Journal of Biological Chemistry, 274(15), 10458-10465.
- Unusual carotenoid composition and a new type of xanthophyll cycle in plants. Proceedings of the National Academy of Sciences, 96(3), 1135-1139.
- The Xanthophyll Cycle Modulates the Kinetics of Nonphotochemical Energy Dissipation in Isolated Light-Harvesting Complexes, Intact Chloroplasts, and Leaves of Spinach. Plant Physiology, 119(2), 531-542.
- . Photosynthesis Research, 62(2/3), 197-203.
- . Photosynthesis Research, 61(1), 77-90.
- Hypothesis: Are grana necessary for regulation of light harvesting?. Functional Plant Biology, 26(7), 659-659.
- Interactions between Senescence and Leaf Orientation Determine in Situ Patterns of Photosynthesis and Photoinhibition in Field-Grown Rice. Plant Physiology, 119(2), 553-564.
- The Relationship between the Binding of Dicyclohexylcarbodiimide and Quenching of Chlorophyll Fluorescence in the Light-Harvesting Proteins of Photosystem II†. Biochemistry, 37(33), 11586-11591.
- Contrasting patterns of photosynthetic acclimation to the light environment are dependent on the differential expression of the responses to altered irradiance and spectral quality. Plant, Cell and Environment, 21(2), 139-148.
- Excited-State Energy Level Does Not Determine the Differential Effect of Violaxanthin and Zeaxanthin on Chlorophyll Fluorescence Quenching in the Isolated Light-Harvesting Complex of Photosystem II. Photochemistry and Photobiology, 68(6), 829-834.
- Ultrafast Evolution of the Excited States in the Chlorophylla/bComplex CP29 from Green Plants Studied by Energy-Selective Pump−Probe Spectroscopy†. Biochemistry, 37(4), 1143-1149.
- Transgenic potato plants with altered expression levels of chloroplast NADP-malate dehydrogenase: interactions between photosynthetic electron transport and malate metabolism in leaves and in isolated intact chloroplasts. Planta, 207(1), 105-114.
- Carotenoid-Dependent Oligomerization of the Major Chlorophyll a/b Light Harvesting Complex of Photosystem II of Plants†. Biochemistry, 36(25), 7855-7859.
- Ultrafast Spectroscopy of Trimeric Light-Harvesting Complex II from Higher Plants. The Journal of Physical Chemistry B, 101(10), 1902-1909.
- Characterisation of LHC II in the aggregated state by linear and circular dichroism spectroscopy. Biochimica et Biophysica Acta (BBA) - Bioenergetics, 1321(1), 61-70.
- Acclimation of photosynthesis to irradiance and spectral quality in British plant species: chlorophyll content, photosynthetic capacity and habitat preference. Plant, Cell and Environment, 20(4), 438-448.
- The xanthophyll cycle and carotenoid-mediated dissipation of excess excitation energy in photosynthesis. Pure and Applied Chemistry, 69(10), 2125-2130.
- Dynamics of Xanthophyll-Cycle Activity in Different Antenna Subcomplexes in the Photosynthetic Membranes of Higher Plants (The Relationship between Zeaxanthin Conversion and Nonphotochemical Fluorescence Quenching). Plant Physiology, 115(4), 1609-1618.
- Identification of proton-active residues in a higher plant light-harvesting complex. Proceedings of the National Academy of Sciences, 93(24), 14204-14209.
- Effect of High Temperature on Photosynthesis in Beans (I. Oxygen Evolution and Chlorophyll Fluorescence). Plant Physiology, 112(3), 1245-1251.
- Effect of High Temperature on Photosynthesis in Beans (II. CO2 Assimilation and Metabolite Contents). Plant Physiology, 112(3), 1253-1260.
- REGULATION OF LIGHT HARVESTING IN GREEN PLANTS. Annual Review of Plant Physiology and Plant Molecular Biology, 47(1), 655-684.
- Dynamic Properties of the Minor Chlorophylla/bBinding Proteins of Photosystem II, anin VitroModel for Photoprotective Energy Dissipation in the Photosynthetic Membrane of Green Plants†. Biochemistry, 35(3), 674-678.
- Quenching of chlorophyll fluorescence in the major light-harvesting complex of photosystem II: a systematic study of the effect of carotenoid structure.. Proceedings of the National Academy of Sciences, 93(4), 1492-1497.
- INFLUENCE OF CHANGES IN THE PHOTON PROTECTIVE ENERGY DISSIPATION ON RED LIGHT-INDUCED DETRAPPING OF THE THERMOLUMINESCENCE Z-BAND. Photochemistry and Photobiology, 62(3), 514-521.
- An Investigation of the Sustained Component of Nonphotochemical Quenching of Chlorophyll Fluorescence in Isolated Chloroplasts and Leaves of Spinach. Plant Physiology, 108(2), 721-726.
- Delayed leaf senescence in ethylene-deficient ACC-oxidase antisense tomato plants: molecular and physiological analysis. The Plant Journal, 7(3), 483-490.
- Resonance Raman Spectroscopy of the Photosystem II Light-Harvesting Complex of Green Plants: A Comparison of Trimeric and Aggregated States. Biochemistry, 34(7), 2333-2337.
- TEMPERATURE DEPENDENCE OF CHLOROPHYLL FLUORESCENCE FROM THE LIGHT HARVESTING COMPLEX II OF HIGHER PLANTS. Photochemistry and Photobiology, 61(2), 216-221.
- Acclimation of Arabidopsis thaliana to the light environment: regulation of chloroplast composition. Planta, 197(3).
- Acclimation of Arabidopsis thaliana to the light environment: changes in photosynthetic function. Planta, 197(2).
- Higher Plant Light-Harvesting Complexes LHCIIa and LHCIIc are Bound by Dicyclohexylcarbodiimide During Inhibition of Energy Dissipation. European Journal of Biochemistry, 226(3), 1063-1069.
- Regulation of Light Harvesting in Green Plants (Indication by Nonphotochemical Quenching of Chlorophyll Fluorescence). Plant Physiology, 106(2), 415-420.
- Invertase: understanding changes in the photosynthetic and carbohydrate metabolism of barley leaves infected with powdery mildew. New Phytologist, 126(2), 213-222.
- Short-term effects of nitrate, nitrite and ammonium assimilation on photosynthesis, carbon partitioning and protein phosphorylation in maize. Planta, 192(2).
- Short-term effects of nitrate, nitrite and ammonium assimilation on photosynthesis, carbon partitioning and protein phosphorylation in maize. Planta, 192(2), 211-220.
- Prompt heat release associated with ΔpH-dependent quenching in spinach thylakoid membranes. Biochimica et Biophysica Acta (BBA) - Bioenergetics, 1185(1), 119-123.
- Modulation of chlorophyll fluorescence quenching in isolated light harvesting complex of Photosystem II. Biochimica et Biophysica Acta (BBA) - Bioenergetics, 1186(1-2), 123-127.
- Acclimation of Arabidopsis thaliana to the light environment: Changes in composition of the photosynthetic apparatus. Planta, 195(2).
- Spectroscopy of non-photochemical and photochemical quenching of chlorophyll fluorescence in leaves; evidence for a role of the light harvesting complex of Photosystem II in the regulation of energy dissipation. Photosynthesis Research, 40(2), 181-190.
- The Effects of Illumination on the Xanthophyll Composition of the Photosystem II Light-Harvesting Complexes of Spinach Thylakoid Membranes. Plant Physiology, 104(1), 227-234.
- Activation of non-photochemical quenching in thylakoids and leaves. Planta, 194(4), 550-556.
- The dissipation of excess excitation energy in British plant species. Plant, Cell and Environment, 16(6), 673-679.
- Relationships between carotenoid composition and growth habit in British plant species. Plant, Cell and Environment, 16(6), 681-686.
- Induction of Nonphotochemical Energy Dissipation and Absorbance Changes in Leaves (Evidence for Changes in the State of the Light-Harvesting System of Photosystem II in Vivo). Plant Physiology, 102(3), 741-750.
- ΔpH-dependent quenching of the Fo level of chlorophyll fluorescence in spinach leaves. Biochimica et Biophysica Acta (BBA) - Bioenergetics, 1142(1-2), 203-206.
- Modulation of ΔpH-dependent nonphotochemical quenching of chlorophyll fluorescence in spinach chloroplasts. Biochimica et Biophysica Acta (BBA) - Bioenergetics, 1183(2), 339-344.
- Excitation-energy quenching in aggregates of the LHC II chlorophyll-protein complex: a time-resolved fluorescence study. Biochimica et Biophysica Acta (BBA) - Bioenergetics, 1141(1), 23-28.
- Aggregation of higher plant xanthophylls: Differences in absorption spectra and in the dependency on solvent polarity. Journal of Photochemistry and Photobiology B: Biology, 21(2-3), 229-234.
- The relationship between Photosystem II intrinsic quantum yield and millisecond luminescence in thylakoids. Photosynthesis Research, 37(2), 131-138.
- Theoretical assessment of alternative mechanisms for non-photochemical quenching of PS II fluorescence in barley leaves. Photosynthesis Research, 36(2), 119-139.
- The molecular mechanism of the control of excitation energy dissipation in chloroplast membranes Inhibition of ΔpH-dependent quenching of chlorophyll fluorescence by dicyclohexylcarbodiimide. FEBS Letters, 309(2), 175-179.
- Mechanisms for controlling balance between light input and utilisation in the salt tolerant alga Dunaliella C9AA. Photosynthesis Research, 32(3), 181-191.
- Light-induced fluorescence quenching and loss of photochemistry in chromatophores of photosynthetic purple bacteria. Journal of Photochemistry and Photobiology B: Biology, 13(3-4), 253-265.
- Mechanism of ΔpH-dependent dissipation of absorbed excitation energy by photosynthetic membranes. II. The relationship between LHCII aggregation in vitro and qE in isolated thylakoids. Biochimica et Biophysica Acta (BBA) - Bioenergetics, 1102(1), 39-44.
- Mechanism of ΔpH-dependent dissipation of absorbed excitation energy by photosynthetic membranes. I. Spectroscopic analysis of isolated light-harvesting complexes. Biochimica et Biophysica Acta (BBA) - Bioenergetics, 1102(1), 30-38.
- pH dependent chlorophyll fluorescence quenching in spinach thylakoids from light treated or dark adapted leaves. Photosynthesis Research, 31(1), 11-19.
- Regulation of Photosystem II. Photosynthesis Research, 34(3), 375-385.
- Control of the light‐harvesting function of chloroplast membranes by aggregation of the LHCII chlorophyll-protein complex. FEBS Letters, 292(1-2), 1-4.
- Resolution of components of non-photochemical chlorophyll fluorescence quenching in barley leaves. Photosynthesis Research, 27(2), 121-133.
- The relationship between zeaxanthin, energy-dependent quenching of chlorophyll fluorescence, and trans-thylakoid pH gradient in isolated chloroplasts. Biochimica et Biophysica Acta (BBA) - Bioenergetics, 1057(3), 320-330.
- Dissipation of excitation energy by Photosystem II particles at low pH. Biochimica et Biophysica Acta (BBA) - Bioenergetics, 1058(2), 187-193.
- Long-wavelength chlorophyll species are associated with amplification of high-energy-state excitation quenching in higher plants. Biochimica et Biophysica Acta (BBA) - Bioenergetics, 1059(3), 355-360.
- The effect of high-energy-state excitation quenching on maximum and dark level chlorophyll fluorescence yield. Photosynthesis Research, 25(3), 199-211.
- Non-photochemical quenching of chlorophyll fluorescence in the green alga Dunaliella. Photosynthesis Research, 24(2), 167-173.
- Uncoupler titration of energy-dependent chlorophyll fluorescence quenching and Photosystem II Photochemical yield in intact pea chloroplasts. Biochimica et Biophysica Acta (BBA) - Bioenergetics, 1016(2), 228-234.
- The mechanisms contributing to photosynthetic control of electron transport by carbon assimilation in leaves. Photosynthesis Research, 25(2), 83-100.
- The mechanisms of changes in Photosystem II efficiency in spinach thylakoids. Biochimica et Biophysica Acta (BBA) - Bioenergetics, 1016(2), 219-227.
- Emerson enhancement, photosynthetic control and protein phosphorylation in isolated maize mesophyll chloroplasts; dependence upon carbon metabolism. Biochimica et Biophysica Acta (BBA) - Bioenergetics, 1017(2), 160-166.
- Regulation of photosystem II by metabolic and environmental factors. Philosophical Transactions of the Royal Society of London. B, Biological Sciences, 323(1216), 269-279.
- Enhancement of the ΔpH-dependent dissipation of excitation energy in spinach chloroplasts by light-activation: correlation with the synthesis of zeaxanthin. FEBS Letters, 256(1-2), 85-90.
- Studies on the induction of chlorophyll fluorescence in isolated barley protoplasts. IV. Resolution of non-photochemical quenching. Biochimica et Biophysica Acta (BBA) - Bioenergetics, 932, 107-115.
- A study of the regulation and function of energy-dependent quenching in pea chloroplasts. Biochimica et Biophysica Acta (BBA) - Bioenergetics, 934(1), 135-143.
- Observation and characterisation of a transient in the yield of chlorophyll fluorescence in intact spinach chloroplasts. Photosynthesis Research, 11(2), 109-118.
- Regulation of thylakoid protein phosphorylation by high-energy-state quenching. FEBS Letters, 221(2), 211-214.
- Characterisation of the effects of Antimycin A upon high energy state quenching of chlorophyll fluorescence (qE) in spinach and pea chloroplasts. Photosynthesis Research, 12(2), 119-127.
- Regulation of photosynthesis in isolated barley protoplasts: the contribution of cyclic photophosphorylation. Biochimica et Biophysica Acta (BBA) - Bioenergetics, 894(2), 332-338.
- Generation of oscillatory behavior in the Laisk model of photosynthetic carbon assimilation. Photosynthesis Research, 12(2), 129-143.
- Studies on the induction of chlorophyll fluorescence in barley protoplasts. III. Correlation betweeen changes in the level of glycerate 3-phosphate and the pattern of fluorescence quenching. Biochimica et Biophysica Acta (BBA) - Bioenergetics, 849(1), 1-6.
- Observation of enhancement and state transitions in isolated intact chloroplasts. Photosynthesis Research, 10(3), 297-302.
- Changes in topography and function of thylakoid membranes following membrane protein phosphorylation. Planta, 168(3), 330-336.
- Heterogeneity in chloroplast photosystem II. Photosynthesis Research, 8(3), 193-207.
- Phosphorylation of chloroplast membrane proteins partially protects against photoinhibition. Planta, 165(1), 37-42.
- DCMU-Induced Fluorescence Changes and Photodestruction of Pigments Associated with an Inhibition of Photosystem I Cyclic Electron Flow. Zeitschrift für Naturforschung C, 39(5), 351-353.
- Increase in the level of thylakoid protein phosphorylation in maize mesophyll chloroplasts by decrease in the transthylakoid pH gradient. FEBS Letters, 176(1), 133-138.
- An investigation of the mechanistic aspects of excitation energy redistribution following thylakoid membrane protein phosphorylation. Biochimica et Biophysica Acta (BBA) - Bioenergetics, 767(3), 568-573.
- An investigation into the ATP requirement for phosphorylation of thylakoid proteins and for the ATP-induced decrease in the yield of chlorophyll fluorescence in chloroplasts at different stages of development. Biochimica et Biophysica Acta (BBA) - Bioenergetics, 767(3), 557-562.
- Phosphorylation of chloroplast thylakoids decreases the maximum capacity of photosystem-II electron transfer. Biochimica et Biophysica Acta (BBA) - Bioenergetics, 767(3), 563-567.
- Studies on the induction of chlorophyll fluorescence in barley protoplasts. II. Resolution of fluorescence quenching by redox state and the transthylakoid pH gradient. Proceedings of the Royal Society of London. Series B. Biological Sciences, 220(1220), 371-382.
- Studies on the induction of chlorophyll fluorescence in barley protoplasts. I. Factors affecting the observation of oscillations in the yield of chlorophyll fluorescence and the rate of oxygen evolution. Proceedings of the Royal Society of London. Series B. Biological Sciences, 220(1220), 361-370.
- Stimulation of a cyclic electron-transfer pathway around photosystem II by phosphorylation of chloroplast thylakoid proteins. FEBS Letters, 162(1), 81-84.
- The influence of metabolic state on the level of phosphorylation of the light-harvesting chlorophyll-protein complex in chloroplasts isolated from maize mesophyll. Biochimica et Biophysica Acta (BBA) - Bioenergetics, 725(1), 155-161.
- Relationships between protein phosphorylation and electron transport in the reconstituted chloroplast system. Biochemical Journal, 210(2), 517-521.
- Characterization of photosystem II electron acceptors in Phormidium laminosum. Archives of Biochemistry and Biophysics, 225(1), 353-359.
- Effects of changes in the capacity for photosynthetic electron transfer and photophosphorylation on the kinetics of fluorescence induction in isolated chloroplasts. Biochimica et Biophysica Acta (BBA) - Bioenergetics, 724(3), 404-410.
- Control of chloroplast electron transport by phosphorylation of thylakoid proteins. FEBS Letters, 152(1), 47-52.
- Relations between electron transport and carbon assimilation; simultaneous measurement of chlorophyll fluorescence, transthylakoid pH gradient and O
2
evolution in isolated chloroplasts. Proceedings of the Royal Society of London. Series B. Biological Sciences, 217(1209), 405-416.
- A comparison between cation and protein phosphorylation effects on the fluorescence induction curve in chloroplasts treated with 3-(3,4-dichlorophenyl)-1,1-dimethylurea. Biochimica et Biophysica Acta (BBA) - Bioenergetics, 722(1), 214-218.
- CHLOROPLAST MEMBRANE PROTEIN PHOSPHORYLATION. Photochemistry and Photobiology, 36(6), 743-748.
- Heterogeneity in Photosystem II. Biochemical Society Transactions, 10(5), 338-340.
- The effect of redox potential on the kinetics of fluorescence induction in Photosystem II particles from Phormidium laminosum. Sigmoidicity, energy transfer and the slow phase. Biochimica et Biophysica Acta (BBA) - Bioenergetics, 680(2), 127-133.
- On the nature of the fluorescence decrease due to phosphorylation of chloroplast membrane proteins. Biochimica et Biophysica Acta (BBA) - Bioenergetics, 680(1), 22-27.
- Light-dependent quenching of chlorophyll fluorescence in pea chloroplasts induced by adenosine 5′-triphosphate. Biochimica et Biophysica Acta (BBA) - Bioenergetics, 635(1), 53-62.
- Does the acceptor Q2
fulfil an indispensable function in the primary reactions of photosystem II?. FEBS Letters, 135(2), 261-264.
- The effect of redox potential of the kinetics of fluorescence induction in pea chloroplasts I. Removal of the slow phase. Biochimica et Biophysica Acta (BBA) - Bioenergetics, 635(1), 105-110.
- Regulation of phosphorylation of chloroplast membrane polypeptides by the redox state of plastoquinone. FEBS Letters, 125(2), 193-196.
- Chloroplast protein phosphorylation and chlorophyll fluorescence quenching. Activation by tetramethyl-p-hydroquinone, an electron donor to plastoquinone. Biochimica et Biophysica Acta (BBA) - Bioenergetics, 638(2), 290-295.
- Light-induced redox changes in chloroplast cytochrome f
after phosphorylation of membrane proteins. FEBS Letters, 132(1), 75-77.
- The effect of redox potential on the kinetics of fluorescence induction in pea chloroplasts. II. Sigmoidicity. Biochimica et Biophysica Acta (BBA) - Bioenergetics, 637(1), 152-158.
- Observation of two quenchers of chlorophyll fluorescence in chloroplasts at −196°C. Biochimica et Biophysica Acta (BBA) - Bioenergetics, 592(3), 559-564.
- Activation of adenosine 5′ triphosphate-induced quenching of chlorophyll fluorescence by reduced plastoquinone. FEBS Letters, 119(1), 141-144.
- Loss of sensitivity to diuron after trypsin digestion of chloroplast photosystem II particles. FEBS Letters, 103(1), 22-26.
- Characterization of two quenchers of chlorophyll fluorescence with different midpoint oxidation-reduction potentials in chloroplasts. Biochimica et Biophysica Acta (BBA) - Bioenergetics, 545(1), 188-201.
- Interactions between Photosystem II components in chloroplast membranes. A correlation between the existence of a low potential species of cytochrome b-559 and low chlorophyll fluorescence in inhibited and developing chloroplasts. Biochimica et Biophysica Acta (BBA) - Bioenergetics, 503(2), 274-286.
- Quenching of chlorophyll fluorescence in chloroplast photosystem II particles by magnesium ions. FEBS Letters, 81(2), 259-263.
- The relationship between the activity of chloroplast Photosystem II and the midpoint oxidation-reduction potential of cytochrome b-559. Biochimica et Biophysica Acta (BBA) - Bioenergetics, 462(1), 86-101.
- Organization and function of chloroplast photosystems. International Journal of Biochemistry, 7(12), 597-605.
- Stimulation of Photosystem I-induced oxidation of chloroplast cytochrome b-559 by preillumination and by low pH. Biochimica et Biophysica Acta (BBA) - Bioenergetics, 430(1), 122-134.
- On the specific site of action of 3-(3,4-dichlorophenyl)-1,1-dimethylurea in chloroplasts: Inhibition of a dark acid-induced decrease in midpoint potential of cytochrome b-559. Archives of Biochemistry and Biophysics, 176(2), 519-524.
- The Effect of ATP on the Photoconversion of Protochlorophyllide in Isolated Etioplasts of Zea mays. Plant Physiology, 56(1), 113-120.
- The Effect of Adenosine 5′-Triphosphate on the Shibata Shift and on Associated Structural Changes in the Conformation of the Prolamellar Body in Isolated Maize Etioplasts. Plant Physiology, 55(2), 393-400.
- RECENT STUDIES ON THE CHLOROPLAST CYTOCHROME b-559. Photochemistry and Photobiology, 22(6), 304-308.
- Acid-base induced redox changes of the chloroplast cytochrome b
-559. FEBS Letters, 56(2), 244-247.
- Light-induced turnover of chloroplast cytochrome b-559 in the presence of N-methylphenazonium methosulphate. Biochimica et Biophysica Acta (BBA) - Bioenergetics, 396(2), 310-319.
- Inhibition of chemical oxidation and reduction of cytochromes ƒ and b-559 by carbonylcyanide p-trifluoromethoxy phenylhydrazone. Biochimica et Biophysica Acta (BBA) - Bioenergetics, 368(3), 361-370.
- The accessibility of the chloroplast cytochromes ƒ and b-559 to ferricyanide. Biochimica et Biophysica Acta (BBA) - Bioenergetics, 368(3), 348-360.
- A chloroplast membrane conformational change activated by electron transport between the region of photosystem II and plastoquinone. Journal of Bioenergetics, 6(4), 167-177.
- Trends in leaf photosynthesis in historical rice varieties developed in the Philippines since 1966. Journal of Experimental Botany, 58(12), 3429-3438.
- Plant immunophilins: functional versatility beyond protein maturation. New Phytologist, 166(3), 753-769.
- Molecular design of the photosystem II light-harvesting antenna: photosynthesis and photoprotection. Journal of Experimental Botany, 56(411), 365-373.
- Efecto de la temperatura, el estrés hídrico y luminoso sobre la heterogeneidad del fotosistema II en cuatro variedades de poroto (Phaseolus vulgaris L.). Revista chilena de historia natural, 74(4).
- Increasing Rice Photosynthesis by Manipulation of the Acclimation and Adaptation to Light, 117-134.
Chapters
- How Protein Disorder Controls Non-Photochemical Fluorescence Quenching, Advances in Photosynthesis and Respiration (pp. 157-185). Springer Netherlands
- Developments in Research on Non-Photochemical Fluorescence Quenching: Emergence of Key Ideas, Theories and Experimental Approaches, Advances in Photosynthesis and Respiration (pp. 73-95). Springer Netherlands
- Dynamic Behavior of Photosystem II Light Harvesting, Encyclopedia of Biological Chemistry (pp. 178-183). Elsevier
- Toward C4 rice: learning from the acclimation of photosynthesis in the C3 leaf, Charting New Pathways to C4 Rice (pp. 333-350). WORLD SCIENTIFIC
- Photosystem II Light Harvesting System: Dynamic Behavior, Encyclopedia of Biological Chemistry (pp. 357-362). Elsevier
- Pressure Induced Fluorescence Quenching in Plant Light Harvesting Complex II, High Pressure Food Science, Bioscience and Chemistry (pp. 417-422). Elsevier
- Photosynthetic Responses of Beans to Water Stress in the Field, Photosynthesis: Mechanisms and Effects (pp. 2561-2564). Springer Netherlands
- All-or-nothing rule for the assembly of photosystem II: an analytical study in severely chlorophyll-deficient tobacco plants, Photosynthesis: Mechanisms and Effects (pp. 3135-3138). Springer Netherlands
- The Effect of PH on Photosynthesis in Photosystem 2 Particles, Current Research in Photosynthesis (pp. 391-394). Springer Netherlands
- Changes in Intrinsic Quantum Yield of Photosystem 2 Observed in Spinach Chloroplasts in vitro, Current Research in Photosynthesis (pp. 399-402). Springer Netherlands
- Uncoupler Titrations of Energy-Dependent Quenching of Chlorophyll Fluorescence in Chloroplasts, Current Research in Photosynthesis (pp. 627-630). Springer Netherlands
- Fluorescence Responses on Step Changes in Irradiance by Plants from Different Light Habitats, Current Research in Photosynthesis (pp. 3155-3158). Springer Netherlands
- The Relationship between the Development of Haustoria of Erysiphe graminis and the Energy Status of Leaves, Current Research in Photosynthesis (pp. 3017-3020). Springer Netherlands
- Regulation of Light Harvesting by Metabolic Events, Current Research in Photosynthesis (pp. 2905-2912). Springer Netherlands
- The Use of Light Pulses to Investigate the Relaxation in the Dark of Chlorophyll Fluorescence Quenching in Barley Leaves, Current Research in Photosynthesis (pp. 631-634). Springer Netherlands
- Photosynthetic Metabolism in Barley Leaves Infected with Powdery Mildew, Current Research in Photosynthesis (pp. 3013-3016). Springer Netherlands
- Photoinhibition of Photosynthetic Bacteria, Current Research in Photosynthesis (pp. 1467-1470). Springer Netherlands
- Photoinhibition of Isolated Chloroplasts and Protoplasts, Progress in Photosynthesis Research (pp. 59-62). Springer Netherlands
- Inhibition of High Energy State Quenching in Spinach Chloroplasts by Low Concentrations of Antimycin A, Progress in Photosynthesis Research (pp. 489-492). Springer Netherlands
- Interplay between Environmental and Metabolic Factors in the Regulation of Electron Transport in Higher Plants, Progress in Photosynthesis Research (pp. 681-688). Springer Netherlands
- Observation of Enhancement and State Transitions in Isolated Intact Chloroplasts, Excitation Energy and Electron Transfer in Photosynthesis (pp. 151-156). Springer Netherlands
- Fluorescence Induction in a Thylakoid System Reconstituted for Photosynthetic Carbon Assimilation, Advances in Photosynthesis Research (pp. 657-660). Springer Netherlands
- Regulation of Light Harvesting Chlorophyll a/b Binding Protein (LHCP) Phosphorylation in Intact Maize Mesophyll Chloroplasts, Advances in Photosynthesis Research (pp. 299-302). Springer Netherlands
- Effects of Protein Phosphorylation on the Properties of Thylakoid Membranes, Advances in Photosynthesis Research (pp. 315-318). Springer Netherlands
- Regulation of the Structure and Function of the Light Harvesting Complexes of Photosystem II by the Xanthophyll Cycle, Advances in Photosynthesis and Respiration (pp. 271-291). Kluwer Academic Publishers
Conference proceedings papers
- Nonphotochemical Quenching of Chlorophyll Fluorescence (pp 99-111)
- Regulation of Non-Photochemical Quenching of Chlorophyll Fluorescence in Plants. Functional Plant Biology, Vol. 22(2) (pp 221-221)
- A sustainable food future. Royal Society Open Science, 10(8).